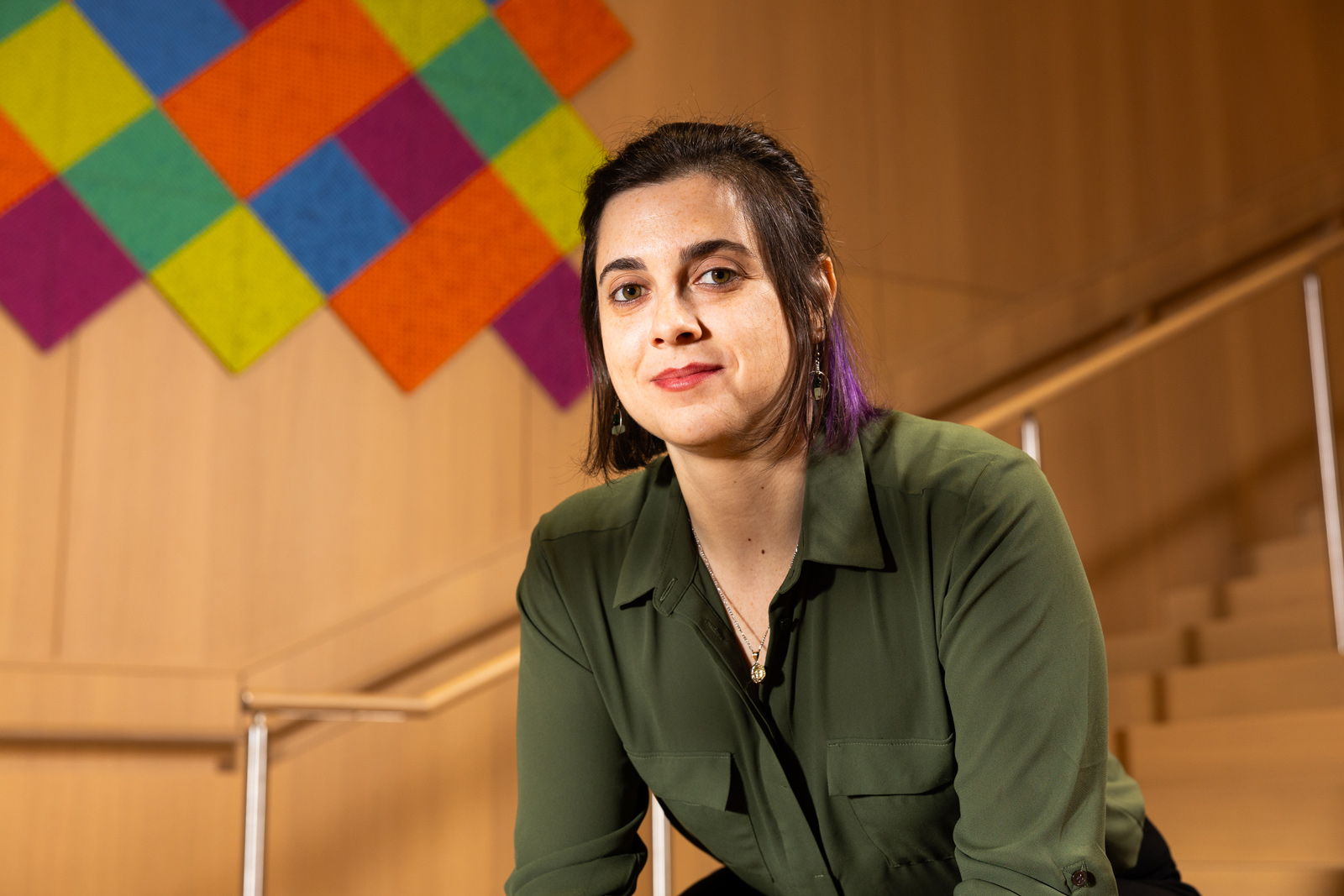
Biometrics in the Age of Artificial Intelligence
Lessons from the past can inform the ethical use of government-sponsored identification systems
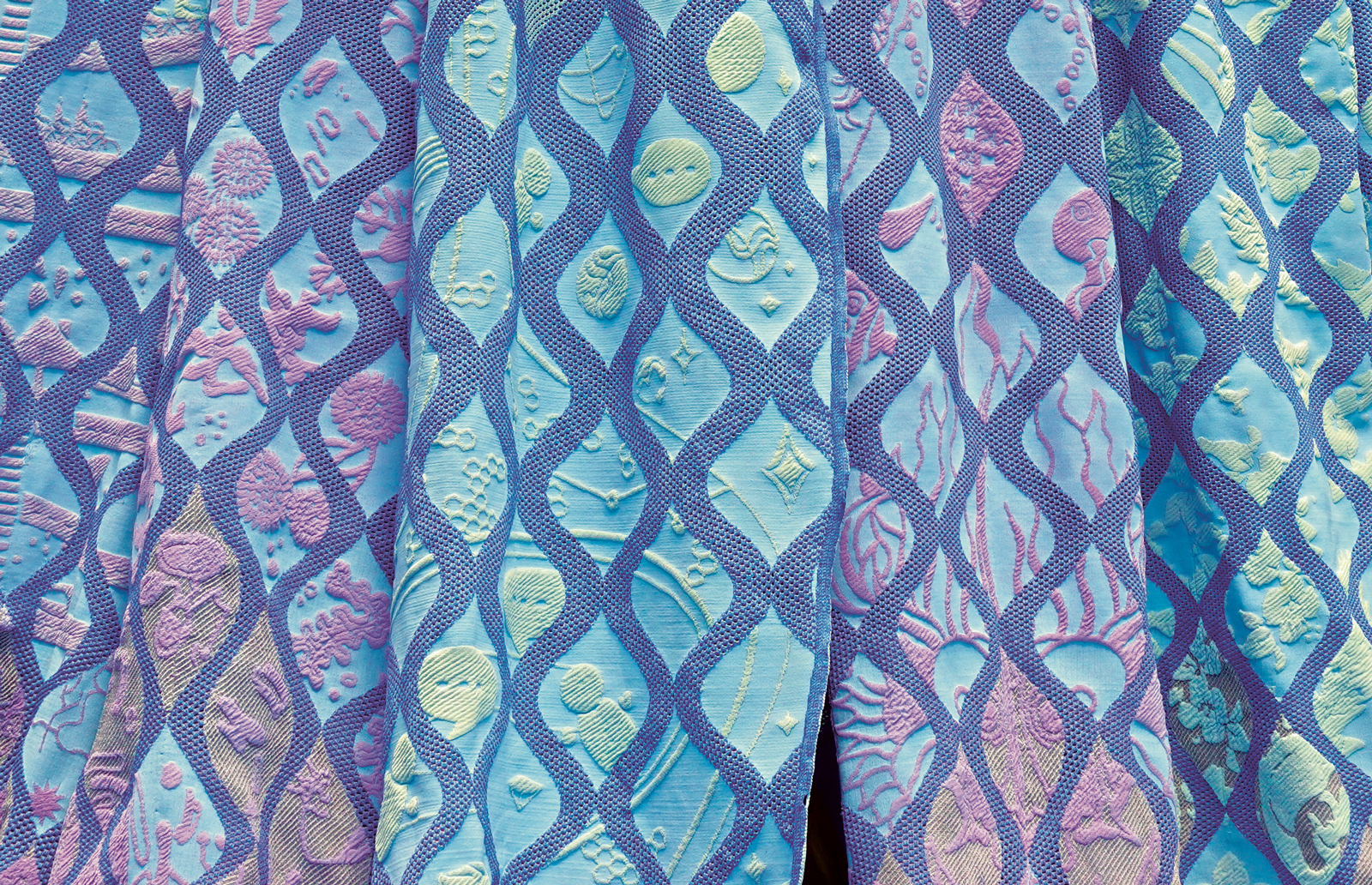
Weaving a Web of Textile Research
MIT Fabric Innovation Hub aims for sustainable clothing manufacturing
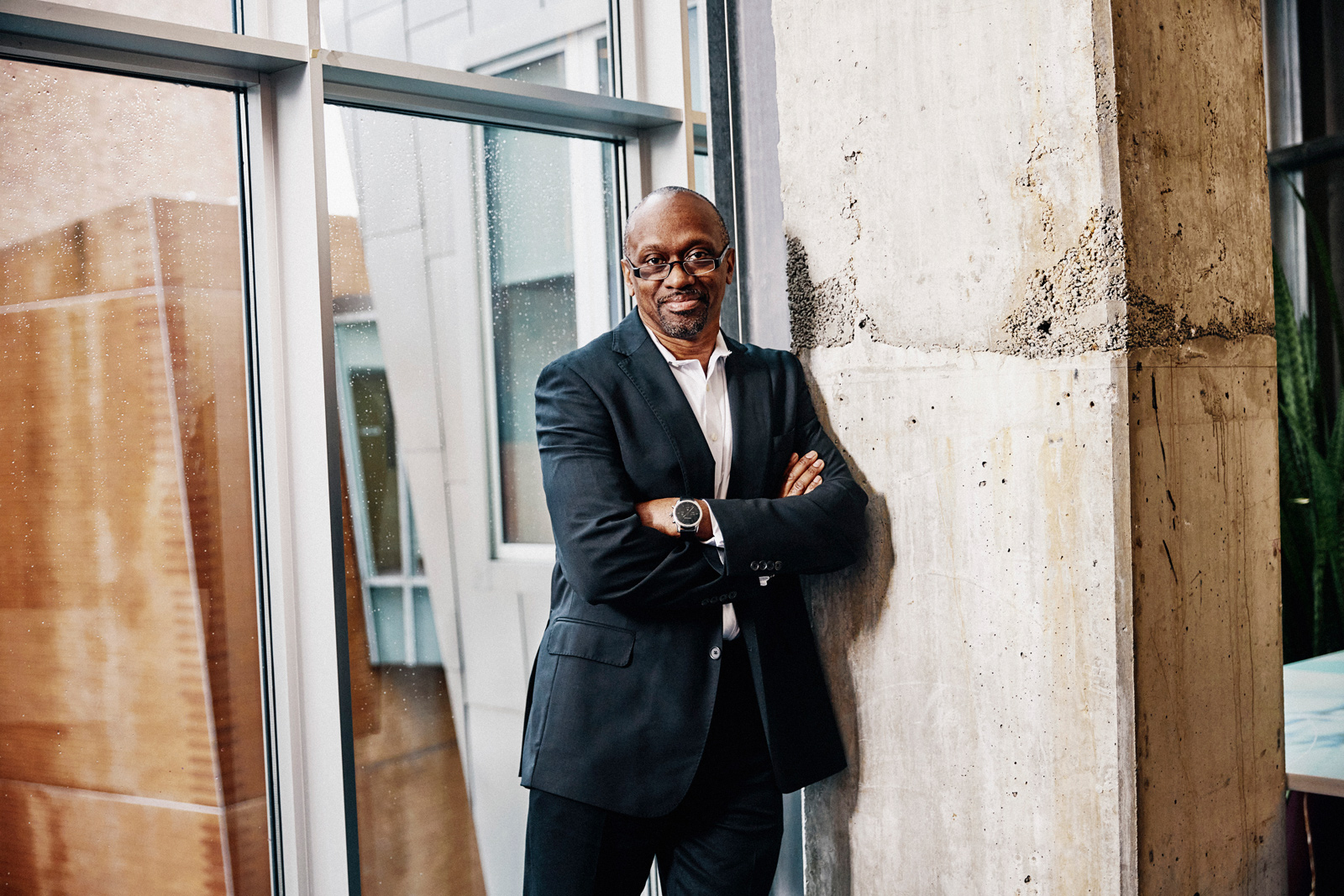
Better Cardiovascular Care through AI
Professor and physician Collin Stultz wants to help heart patients everywhere by applying machine-learning techniques to cardiovascular medicine
MIT’s Responsibility to Lead in a Challenging World
Vice president for resource development talks about the Institute’s vital role in addressing the most critical issues of our time
Economics Research to Change the World
New fundraising effort keeps MIT Department of Economics at the vanguard of an evolving field
Our Fandoms, Ourselves
In Comparative Media Studies/Writing class, students explore fan culture as “a powerful tool for social engineering”
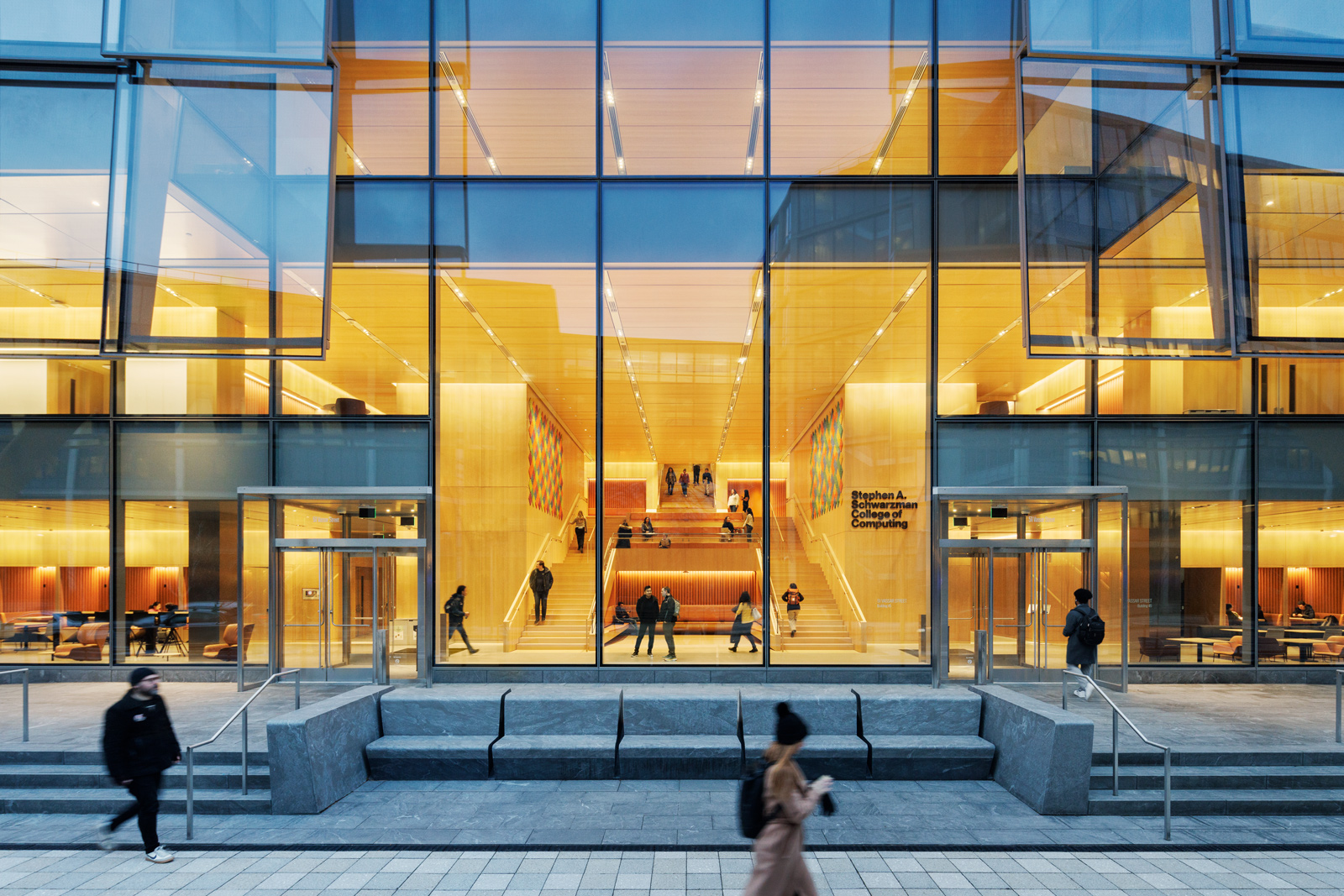
A State-of-the-Art Space for Sparking Innovation in Computing and AI
The new home of MIT’s Stephen A. Schwarzman College of Computing opens the college to the world
Spotlight On: What's Next?
New Faculty at the MIT Schwarzman College of Computing
The MIT Schwarzman College of Computing is fulfilling its commitment to create 50 new faculty positions
MIT Sloan research helps family enterprise investors amplify their impact with a systems approach
Polymers for People and the Planet
Researcher's pioneering work in polymeric chemistry and chemical engineering yields materials for highly targeted cancer treatments and sustainable plastics
Charles Stewart III explores the way Americans vote, in all its extraordinary complexity
Popular
A Robotic Helping Hand for Aging in Place
Mechanical engineering professor Harry Asada works on tools that may be able to help elderly people retain their independence
Technical policy scholar Patrick White joins the SPARC project to ask: what comes after success?
D-Lab Marks 20 Years of Empowering Communities
Academic program puts tools of design into action around the world